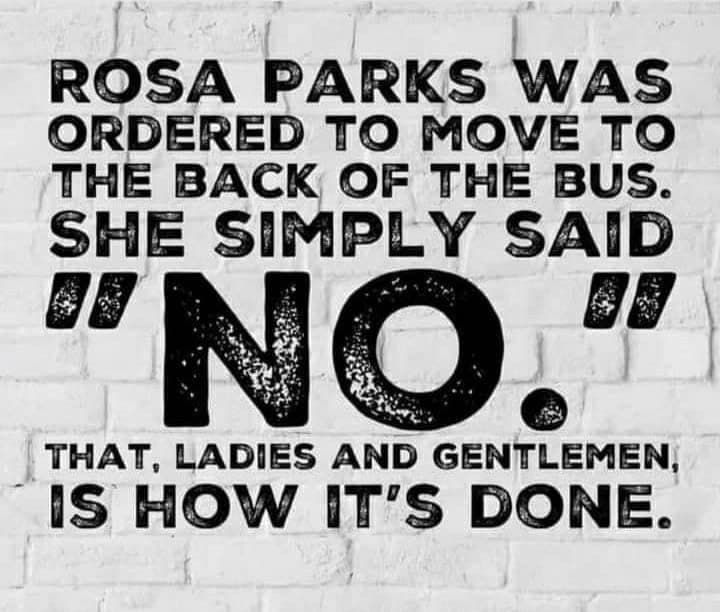
Shedding Study Spike & mRNA
HYPOTHESIS
Infectious Diseases Research 2022;3(4):22. https://doi.org/10.53388/IDR20221125022
Submit a manuscript: https://www.tmrjournals.com/idr 1
Current state of knowledge on the excretion of mRNA and spike produced by
anti-COVID-19 mRNA vaccines; possibility of contamination of the entourage of
those vaccinated by these products
Helene Banoun1*
1Pharmacist biologist, Former Inserm researcher, Member of the Independent Scientific Council, Marseille 13000, France.
*Corresponding to: Helene Banoun, Pharmacist biologist, Former Inserm researcher, Member of the Independent Scientific Council, Marseille 13000, France.
E-mail: helene.banoun@laposte.fr.
Competing interests
The author declares no conflicts of interest.
Acknowledgments
This research received no specific grant from any funding
agency in the public, commercial, or not-for-profit sectors.
Abbreviations
LNPs, lipid nanoparticles; MMR, measles/mumps/rubella; EVs,
extracellular vesicles; VEGF, vascular endothelial growth
factor; pDNA, plasmid DNA; IM, intramuscular; VLP, virus like
particles; RBD, receptor binding domain (spike).
Citation
Banoun H. Current state of knowledge on the excretion of
mRNA and spike produced by anti-COVID-19 mRNA vaccines;
possibility of contamination of the entourage of those
vaccinated by these products. Infect Dis Res. 2022;3(4):22. doi:
10.53388/IDR20221125022.
Executive editor: Na Liu.
Received: 11 October 2022; Accepted: 07 November 2022;
Available online: 14 November 2022.
© 2022 By Author(s). Published by TMR Publishing Group
Limited. This is an open access article under the CC-BY license.
(https://creativecommons.org/licenses/by/4.0/)
Abstract
The massive COVID-19 vaccination campaign is the first time that mRNA vaccines have
been used on a global scale. The mRNA vaccines correspond exactly to the definition of gene
therapy of the American and European regulatory agencies. The regulations require
excretion studies of these drugs and their products (the translated proteins). These studies
have not been done for mRNA vaccines (nor for adenovirus vaccines). There are numerous
reports of symptoms and pathologies identical to the adverse effects of mRNA vaccines in
unvaccinated persons in contact with freshly vaccinated persons. It is therefore important to
review the state of knowledge on the possible excretion of vaccine nanoparticles as well as
mRNA and its product, the spike protein.
Vaccine mRNA-carrying lipid nanoparticles spread after injection throughout the body
according to available animal studies and vaccine mRNA (naked or in nanoparticles or in
natural exosomes) is found in the bloodstream as well as vaccine spike in free form or
encapsulated in exosomes (shown in human studies). Lipid nanoparticles (or their natural
equivalent, exosomes or extracellular vesicles (EVs)) have been shown to be able to be
excreted through body fluids (sweat, sputum, breast milk) and to pass the transplacental
barrier. These EVs are also able to penetrate by inhalation and through the skin (healthy or
injured) as well as orally through breast milk (and why not during sexual intercourse
through semen, as this has not been studied). It is urgent to enforce the legislation on gene
therapy that applies to mRNA vaccines and to carry out studies on this subject while the
generalization of mRNA vaccines is being considered.
Keywords: COVID-19 vaccine; vaccine shedding; COVID vaccine adverse effects; Lipid
nanoparticles; LNPs; mRNA vaccine; exosome; exosome excretion route; gene therapy; spike
protein; LNPs excretion routes; exosomes penetration
HYPOTHESIS
Infectious Diseases Research 2022;3(4):22. https://doi.org/10.53388/IDR20221125022
Submit a manuscript: https://www.tmrjournals.com/idr 2
Introduction
Why are we interested in this hypothesis, which may seem
conspiracist?
The expression “vaccine shedding” classically refers to the possible
excretion of a virus by a person who has been freshly vaccinated
against that virus; this is valid only for live attenuated virus vaccines
(measles/mumps/rubella (MMR), chickenpox, rotavirus, nasal spray
influenza).
No COVID-19 vaccine uses this formula. Therefore, there is no risk
that a vaccine recipient will transmit a vaccine virus. However,
mRNA-based COVID-19 vaccines are the first to be used commercially
in humans on a global scale and no studies have been conducted
regarding the possible excretion of the vaccine itself (lipid
nanoparticles containing mRNA) of the vaccine mRNA or of the
vaccine product, the spike protein translated by the cells of the
vaccinee.
The COVID vaccination started in December 2020. The first
published testimony of vaccine shedding that I saw dates from
December 2021 and is that of Dr Ray Sahelian [1]: he reported cases
of medical or scientific colleagues who had observed symptoms close
to those of the adverse effects of the vaccine after having been in
contact with freshly vaccinated persons; he proposed an excretion of
the products of the vaccine by the skin and the respiratory tract and
asked for complementary studies.
At the beginning, this type of testimony did not seem very credible
to me, but they accumulated and in October 2021, I received a
testimony from a group of French caregivers: they observed a stroke in
a 7-year-old child with no risk factors and whose parents had been
freshly vaccinated. There are Telegram groups listing testimonies from
patients and doctors. All of these testimonials report symptoms or
conditions reported in the COVD-19 vaccine adverse event databases :
the adverse effects of mRNA vaccines against COVID-19 are now
recognized by regulatory agencies (see VAERS and Eudravigilance
databases, as well as the ANSM, France).
The vaccines are all based on the spike protein, which has since
been recognized as the main responsible for the pathogenicity of
SARS-CoV-2 [2-6]. Therefore, in the event that the vaccine or its
product (the spike) passes from vaccinated to unvaccinated, the
adverse effects of the vaccine should be found in some unvaccinated
people in contact with vaccinated people. The exploration of
vaccine-related pathologies in non-vaccinated age groups in contact
with vaccinated people could give indications in the sense of vaccine
shedding, but it does not give significant results (unpublished). As
there are more than 400 pathologies related to adverse vaccine
reactions in the pharmacovigilance reporting databases (see for
example, the UK data, spontaneous notification data for Pfizer vaccine
in May 2021 [7]), this large number dilutes the signals that could
appear in non-vaccinated age groups.
On the other hand, an analysis of European, Israeli and US data
shows that for the non-vaccinated 0-14 age group, most of the
associations between mortality and vaccination in adults are positive:
the excess mortality in non-vaccinated age groups when vaccination
campaigns begin could be explained by a transmission phenomenon of
the vaccine or its products. This pattern of positive correlations
increases from the week of vaccination to week 18 after vaccination
and then disappears. It indicates indirect negative effects of adult
vaccination on mortality in children aged 0-14 years during the first
18 weeks after vaccination [8].
What is the biological plausibility of transmission of the vaccine
or its products from vaccinated to unvaccinated?
To answer this question, we need to explore the possibility and routes
of excretion of the vaccine or its products and the routes of their
possible penetration.
Concerning the vaccine and its products, it may be the transmission
of the circulating spike in the vaccinated (in free form or included in
exosomes or EVs), the transmission of circulating naked mRNA or
complete lipid nanoparticles (LNPs).
Therefore, the ability of LNPs, mRNA and vaccine spike to be
excreted by different possible routes and then the ability of the same
products to enter by different routes into the body of unvaccinated in
close contact with vaccinated should be explored.
The excretion of mRNA-containing LNPs, the excretion of modified
spike-encoding mRNA, and the excretion of spike produced by
vaccinees have not been studied in the trial phase of the vaccines,
contrary to the recommendations of the regulators concerning gene
therapies. Pharmacokinetic studies of nanoparticles in general have
not explored the excretion of the transporters or the transported
molecules. This area should be explored.
Pfizer documents obtained by FOIA [9] show that only the excretion
of some components of the LNPs (ALC-0315 and ALC-0159) was
studied in the urine and feces of IM injected rats.
Regulations regarding the excretion of gene therapies by regulatory
agencies
There was no regulation of mRNA clinical trials prior to RNA vaccines,
yet there is strict regulation of gene therapy products. It is difficult to
justify that mRNA vaccines are not considered in the same way as
gene therapies regarding this regulation; indeed the only difference is
that they are supposed to protect against a disease and not cure it.
Gene therapies are intended for a small number of people in poor
health, whereas vaccines are used on a large scale on healthy people:
it would therefore be wise to apply stricter rules to them. However,
the description of gene therapy products provided by the regulatory
agencies does include mRNA and adenovirus vaccines.
The 2015 FDA document on Gene Product Shedding Studies [10]
concerns gene therapies, which are defined as “all products that exert
their effects by transcription and/or translation of transferred genetic
material and/or by integration into the host genome and that are
administered in the form of nucleic acids, viruses or genetically
modified microorganisms”. In this sense mRNA vaccines are indeed
gene therapy products and should have been submitted to these
excretion studies.
Excretion studies must be conducted for each VBGT (virus or
bacteria-based gene therapy products), first in animals but also in
humans, especially when there is a risk of transmission to untreated
individuals. According to this document, clinical excretion studies are
not stand-alone studies but are integrated into the design of a safety or
efficacy trial. The term “shedding” refers to the release of VBGT
products from the patient by any or all of the following routes: feces
(feces); secretions (urine, saliva, nasopharyngeal fluids, etc.); or
through the skin (pustules, lesions, sores).
The NIH guidelines [11] provide biosafety principles specifically for
“synthetic nucleic acid molecules, including those that are chemically
or otherwise modified but can pair with naturally occurring nucleic
acid molecules”; these are molecules of more than 100 nucleotides
with the potential to be transcribed or translated. This April 2019
document is about modified and unmodified synthetic nucleic acids.
Any experiment involving the deliberate transfer of a nucleic acid to a
human must be preceded by Institutional Biosafety Committee
approval (which is confirmed here [12]), but approval was not given
because of the emergency clearance given to mRNA vaccines.
Based on an EMA document on excretion of gene products [13],
mRNA vaccines meet the definition of GMTPs (gene therapy medicinal
products), however their designation as a “vaccine” has allowed them
to escape the clinical trial requirements for gene products that relate
in particular to excretion potential, biodistribution,
pharmacodynamics, genotoxicity, insertional mutagenesis (page 36 :
Pharmacokinetic studies should be performed when a protein is
excreted into the bloodstream). The expression of the nucleic acid
sequence (its translation into protein) should also be studied (page
37). Excretion is defined as the dissemination of the vector through
secretions and/or feces and should be addressed in animal models
(page 30).
Therefore, according to the regulations of the American and
European agencies, mRNA vaccines correspond to the definition of
HYPOTHESIS
Infectious Diseases Research 2022;3(4):22. https://doi.org/10.53388/IDR20221125022
Submit a manuscript: https://www.tmrjournals.com/idr 3
gene therapy products and should have been subjected to excretion
studies by all secreted fluids (urine, saliva, sputum, nasopharyngeal
fluids, semen, breast milk), feces and skin (healthy or injured). These
studies should have concerned the nanoparticles containing the
mRNA, the naked mRNA and the product of the vaccine after
translation (the spike protein).
An example of an excretion study corresponding to this regulation
of gene products can be found in a report submitted to the EMA to
authorize a drug intended to treat an orphan disease; it is a product
based on LNPs with a composition close to that of mRNA vaccines.
Here the LNPs contain siRNA. The regulations require extensive
studies for this gene therapy, unlike those for mRNA vaccines, which
are similar. However, studies on the excretion of these LNPs give little
information. In animals, the radioactivity of LNPs is found in the urine
(50%) and in the feces (between 10% and 24%). In humans, no study
with radioactive LNPs has been performed, but the components of
LNPs are found in the urine for less than 1% of the plasma
concentrations. It is assumed that elimination is via the feces but this
has not been proven. There have been no studies on excretion in milk
or other body fluids [14].
Reference to possible vaccine shedding in Pfizer documents
The protocol for the Pfizer Phase I/II/III trial of COVID-19 mRNA
vaccines (which began in May 2020) mentions the possibility of
passage of the study product through inhalation or skin contact and
passage through semen from a man exposed through inhalation or
skin contact and passage through breast milk; the possibility of an
adverse vaccine reaction from these exposures is also mentioned [15].
Pfizer's data clearly indicate that a pregnant woman may be exposed
to “the intervention studied due to environmental exposure.”
Environmental exposure can occur through “inhalation or skin
contact.” Examples of environmental exposure during pregnancy
include: -A female family member or health care provider reports that
she is pregnant after being exposed to the study intervention through
inhalation or skin contact. -A male family member or health care
provider who was exposed to the study intervention by inhalation or
skin contact subsequently exposes his female partner before or around
the time of conception. This clearly means that any contact, including
sexual contact with someone who has received the vaccines, exposes
those who have not received the vaccines to the “intervention”, i.e.
mRNA. Exposure during breastfeeding had also to be immediately
notified during the trial: it is assumed that the investigator is
concerned that a breastfeeding mother could transmit the
experimental mRNA to her baby if she received the vaccines directly
or if she is “exposed to the study intervention by inhalation or skin
contact.”
Structure and function of extracellular vesicles (EVs) or exosomes
and lipid nanoparticles (LNPs)
Natural extracellular vesicles (EVs or exosomes) are generated by most
living cells, they are spherical bilayer proteolipids ranging in size from
20 to 4,000 nm and they can contain various molecules (lipids,
proteins and nucleic acids, like signaling RNAs). EVs are natural
carriers in the human body and are involved in intercellular
communications, they can serve as transporters for different molecules
that can thus pass from cell to cell, resulting in a marked response
from the target cell [16]. Synthetic mRNA vaccine LNPs have the same
structure as the natural exosomes they seek to mimic [17, 18].
Naturally produced exosomes can carry spike or vaccine mRNA as
discussed below. LNPs have the ability (like natural exosomes) to fuse
with cell membranes and release their cargo into the cytosol.
LNPs used for mRNA vaccines are nanosized (less than 1
micrometer) lipid systems made of 2 or more (usually 4) lipids at
varying ratios. The most typical lipid composition used for mRNA-LNP
systems consists of a cationic/ionizable lipid, a phospholipid “helper
lipid”, cholesterol and/or a poly(ethylene glycol) (PEG) associated
lipid. LNPs can be administered IM, subcutaneously, intradermally,
intratracheally, orally, ophthalmically and even topically. LNP
injected by all of these routes is capable of driving translation of
mRNA to protein for several days [19]. The size of LNPs in COVID-19
mRNA vaccines is reported to be between 60 and 100 nm [20].
This trafficking of EVs is bidirectional during pregnancy (EVs cross
the fetomaternal barrier and uterine cells constantly secrete
exosomes) and EVs can be used to deliver drugs to the fetus during
pregnancy [21].
EVs have a potential advantage for use in vaccine therapies because
they are the body's natural antigen carriers and can circulate in body
fluids to distribute antigens even to distal organs [16].
Little is known about the pharmacokinetics of mRNA vaccines
Nanoparticles in animals
According to a study by researchers independent of mRNA vaccine
manufacturers, in mice, mRNA-carrying LNPs injected IM pass from
the injection site into the lymph nodes and then into the systemic
circulation, accumulating primarily in the liver and spleen. LNPs pass
first into the lymphatic circulation and then into the bloodstream
(LNPs smaller than 200 nm pass directly into the lymph, while those
between 200 and 500 nm are transported into the lymph by dendritic
cells). Unintentional direct injection into a blood vessel may also
occur during intramuscular (IM) injection [22].
Nanoparticles in humans
Exposure of the human body to nanoparticles can occur accidentally
through inhalation, skin contact, or ingestion. In the case of
inhalation, the possible routes of transfer of nanoparticles are the
bloodstream (systemic), the lymphatic vessels, the gastrointestinal
tract and the central and/or peripheral nervous system [23].
Excretion of PEG-coated LNPs is primarily through feces and urine
and primarily through feces when they are > 80 nm in diameter.
LNPs can be excreted through saliva, sweat, and breast milk [24].
LNPs of size < 5 nm are rapidly excreted by the kidney.
Nanoparticles that are between 5 and 200 nm tend to have extensive
blood circulation. Larger LNPs have prolonged blood circulation and
little renal excretion. Because of the size of LNPs, inhalation is the
most direct route of entry into the pulmonary system. Exposure can be
intentional, as in the case of targeting or therapeutic nanoparticles, or
unintentional, through inhalation or dermal exposure, due to the
increasing number of industrial applications of nanoparticles [25].
The mRNA
Persistence of viral mRNA after viral infections. The viral RNA of
some viruses persists for a long time in the brain, the eyes, the
testicles: this has ben showed for the measles virus, the Ebola virus,
Zika and Marburg. SARS-CoV-2 persists in the respiratory tract and
intestine. Viral RNAs are also detected in secretions, blood, or tissue.
Prolonged shedding of these RNAs in the respiratory tract, feces,
sweat, conjunctival fluid, and urine is common. Studies have shown
that full-length viral RNA can persist over the long term. This
persistent RNA can be translated into protein even if no viable virus
can be assembled.
In patients who later develop long COVID, viral RNA is found in the
blood in the acute phase of the disease [26].
Fate of vaccine mRNA. Huge amounts of mRNA are injected
compared to the circulation of a virus during a natural infection: up to
10 to 7 times more, according to Professor Jean-Michel Claverie [27].
Vaccine mRNA is present from day one and persists in the
bloodstream for at least 2 weeks after injection; its concentration
starts to decrease after 4 days. This lifetime is much longer than was
claimed by the manufacturers on the basis of brief studies in rats. The
transported mRNA is encapsulated in LNPs but is found in plasma (i.e.
not associated with white blood cells). This mRNA is capable of being
translated into spike protein in susceptible cells and tissues [28].
mRNA packaged in LNPs is able to escape from LNPs and form
extracellular vesicles that transport it to other cells: these vesicles are
secreted after endocytosis of mRNA-loaded LNPs. These EVs protect
HYPOTHESIS
Infectious Diseases Research 2022;3(4):22. https://doi.org/10.53388/IDR20221125022
Submit a manuscript: https://www.tmrjournals.com/idr 4
the mRNA during transport and distribute it intact to the recipient
cells, the mRNA is functional and can then be translated into the
protein of interest. The inflammatory response is lower after
transfection with EVs than with LNPs. The uptake pathways of EVs
differ from those of LNPs and are not likely to trigger the
autophagic-lysosomal pathway, as they release their contents into the
cytoplasm presumably without undergoing lysosomal trapping.
Moreover, because of their small size, EVs can escape rapid
phagocytosis and routinely transport and deliver RNA into the
circulation, crossing the vascular endothelium to target cells [29].
The presence of extracellular vesicles in all biofluids is attested.
They can contain nucleic acids. In sweat, we find EVs containing
nucleic acids from bacteria, viruses, skin fungi but also from human
cells. These EVs can also contain viruses (hepatitis C, for example).
Small mRNAs (20 to 200 bp) are found in these sweat EVs; they are
functional (can be translated), RNAs are protected from skin nucleases
in the EVs [30].
Note that the vaccine RNA comprises 4,284 nucleotides (Pfizer)
[31]. Thus, the possibility of RNAs of this size being excreted through
sweat should be explored.
EVs may contain “signal” molecules such as miRNAs. It is possible
that EVs contain full-length mRNAs, which are key mediators of
intracellular communication. Blood and sweat RNA analyses are
correlated: the EVs found in sweat reflect the circulation of EVs in
plasma. Bare RNAs are also found in sweat (not encapsulated in EVs).
MiRNAs are selectively selected and enriched in sweat EVs from blood
and do not passively circulate in any blood or sweat fractions [32].
An increase in sweating after the COVID vaccine has been noted
[33] and people who have received the vaccine have complained of
increased sweating, particularly at night [34].
The possibility of exudation of extracellular vesicles from the skin
has been shown: keratinocytes are able to exude extracellular vesicles
capable of carrying miRNAs. In psoriasis, EVs excreted by
keratinocytes pass from cell to cell: from keratinocyte to neighboring
keratinocyte. In patients with lichen planus (inflammatory rash)
extracellular vesicles carrying miRNAs are excreted in saliva [35].
Nanoparticles are naturally present in sputum [36]: RNA-containing
exosomes were isolated from sputum of mild asthmatic patients [37].
Passage of vaccine mRNA into milk. Vaccine mRNA was found in
the milk of 1/10 women studied (4/40) in the first week after
vaccination with mRNA vaccine (either after dose 1 or dose 2).
Amounts can reach 2 ng/mL of milk [38]. This amount may seem
small compared to the 30 micrograms of mRNA injected with the
vaccine, but it can be enough to produce a significant amount of spike.
Indeed, an infant makes several feedings per day, for approximately
240 to 360 mL per day and a total over a week of 1680 to 2,520 mL in
the first week. The newborn, weighing between 2 and 5 kg, could
therefore be exposed to a dose of 5 μg of mRNA in its first week. This
seems disproportionate compared to the 10 μg injected into children
aged 5 to 11 years who weigh approximately 18 to 35 kg respectively
[39]. The method used in the latter study is more sensitive than that of
Golan et al. who did not find mRNA in milk [40]. This same team had
also explored the passage of vaccine mRNA into milk by indirectly
searching for PEG contained in LNPs. PEG was searched for in the milk
of 13 women at varying times after vaccination: Figure 1 of the article
shows the detection of vaccine PEG in milk between 24 hours and one
week after injection. However, the authors concluded without
specifying that these quantities were not significant [41].
Another study investigated whether COVID-19 vaccine mRNA can
be detected in the expressed breast milk of breastfeeding individuals
who received vaccination within 6 months of delivery. The presence
of mRNA was investigated in free form and encapsulated in
extracellular vesicles. EVs were isolated by centrifugation of milk.
Vaccine RNA was found within 48 h of vaccination and in higher
concentrations in EVs than in whole milk. The highest concentration
found was 17 pg/mL in EVs and the lowest was 1.3 pg/mL in whole
milk. The priority presence of mRNA in EVs and not in whole milk
may explain why Golan et al. did not find it [42].
It has been known for some years that mRNA encapsulated in
extracellular vesicles is protected from gastric juices and can transfect
intestinal cells [43, 44]. A recent review by Melnik and Schmitz
confirms that milk EVs survive the extreme conditions of the
gastrointestinal tract, are internalized by endocytosis, are
bioavailable, reach the bloodstream, and penetrate peripheral tissue
cells [45].
Transplacental passage of nanoparticles? In mice, LNPs of the same
type as those used in COVID-19 mRNA vaccines have shown their
ability to transfect mRNA after injection into a fetal vein or in utero
[46].
In an attempt to immunize fetuses against neonatal herpes in
pregnant mice by injection of mRNA-loaded LNPs into the mother, the
possibility that transplacental passage of LNPs would explain both
fetal immunization and maternal passage of induced Ig is not
discussed [47].
Studies have shown that it is very possible that nanoparticles of
comparable size to those used for mRNA vaccines are capable of
transplacental passage in humans [48, 49].
The delivery of LNP-based therapies during pregnancy poses risks
that should be investigated. Detection of transplacental passage
depends on the sensitivity of the detection methods: for some types of
nanoparticles embryotoxicity has been observed while no absorption
by the fetus was observed; this absorption does not seem to correlate
with the type, size or surface electric charge of the nanoparticles.
Translocation of nanoparticles is likely to depend on the different
stages of pregnancy. During the first trimester, the placental barrier is
very thick to protect the developing embryo and becomes thin at term
when large amounts of nutrients are needed to support fetal growth.
However, in animals, placental transfer appears to be higher in early
pregnancy. There is a need to develop human models for NP transfer
studies in early pregnancy. Comparison with animal studies is
essential, as the placenta is the most species-specific organ [50, 51].
240 nm nanoparticles are able to cross the human placental barrier
[52].
All these publications underline the difficulty of extrapolating
animal studies to humans concerning the transplacental passage of
nanoparticles. From a 2022 review [53], nanoparticles can transit
through ordinary placental transcellular transport mechanisms such as
pinocytosis, active transport, facilitated diffusion and passive
diffusion. RNA cargo exosomes are also able to cross the human
placental barrier. PEG-coated LNPs are reported to have less diffusion
across the placental barrier than liposome-based formulations, but are
able to deliver some of their cargo to the fetus [54].
All of these data cannot rule out that LNPs from mRNA vaccines are
capable of reaching the fetus of a vaccinated mother during
pregnancy.
Excretion of LNPs into the sperm? I have not found any studies
regarding the possibility of LNPs passing into the sperm; however, the
effect of nanoparticles on fertility and sperm quality has been widely
studied in animals [55]. The toxicity of nanoparticles on male
reproductive function is well established, gold nanoparticles have
been shown to act only by interacting with the surface of sperm cells
but not penetrating them. No data is available on the possible
penetration of LNPs into the sperm.
According to a confidential Pfizer document obtained through the
FOIA [56] concerning pharmacokinetic studies in rats, LNPs
concentrate in the ovaries and to a lesser extent in the testes.
HYPOTHESIS
Infectious Diseases Research 2022;3(4):22. https://doi.org/10.53388/IDR20221125022
Submit a manuscript: https://www.tmrjournals.com/idr 5
Figure 1 State of knowledge on excretion of mRNA vaccines
Fate of spike protein after mRNA translation
A CDC-sponsored news site accessed on July 21, 2021 notes that the
lifespan of spike in the bloodstream is “unknown and may be a few
weeks.” [57]. Injection of LNPs containing pseudouridine-modified
mRNA by IM, subcutaneously and intradermally results in protein
production at the site of injection, the duration of active translation is
6 to 10 days in mice. Intradermal injection produces a lower initial
amount of protein but over a longer period of time than the IM route.
By the intradermal route, the half-life of protein production is the
longest compared with other injection routes (IM, subcutaneous, IV,
Iperitoneal, intra-tracheal). By IM delivery, the majority of translation
ceased in the liver at day 2 post-injection but lasted for up to 8 days in
muscles [58].
In humans, the spike protein could persist for a long time in
vaccinees, monitoring of vaccine adverse effects should therefore be
extended [59]. Comparison of spike concentrations achieved during
disease and after vaccination shows that during severe COVID-19 the
median concentration observed is 50 pg/mL with maximums at 1
ng/mL. During severe Covid infection, levels of up to 135 pg/mL of S1
spike can be detected, most commonly between 6 and 50 pg/mL. After
vaccination with mRNA vaccine concentrations up to 150 pg/mL are
commonly observed but may reach 10 ng/mL in individuals with
vaccine-induced thrombocytopenia [60].
The same team [61] also shows that spike protein persists for a long
time in free form: vaccine-induced spike mRNA circulates in plasma as
early as D1 after vaccination and up to 14 days, with the peak
occurring at D5 with 68 pg/mL of S1 sub-unity detected; full-length
spike is detected up to D15, with a peak at 62 pg/mL. After the 2nd
dose, free spike is no longer detected as it would be bound to
antibodies; the study does not detect antibody-spike immune
complexes.
Another team also showed that, after vaccination with mRNA, spike
protein enters the bloodstream, persists for more than a week and is
completely eliminated within 1 month. The increase in blood spike
concentration after vaccination is rapid (1 to 3 days) [62].
According to an autopsy, vaccine spike is found up to three weeks
after injection in different organs (heart, brain, muscles, germinal
centers, etc.) and particularly in the endothelium of capillaries [63].
Circulating spike-containing exosomes
After COVID-19 infection, spike circulates as exosomes. Exosomes are
released from cells into the extracellular environment under normal
and pathological conditions. Exosomes are an important tool for
intercellular communication, as they serve as shuttles for the transfer
of biologically active proteins, lipids, and RNA. EVs can incorporate
pathogenic proteins and/or viral RNA fragments from infected cells to
mRNA Vaccine
= mRNA in LNPs
Biodistribution
LNPs
Bloodstream,Lymph
CNS, Testes,
Concentration in
liver, spleen, ovaries
mRNA
naked or encapsulated
in natural EVs
2 weeks in bloodstream
Translation
mRNA > spike protein
at the injection site in
mice: lasts 6 to 10 days
Naked spike circulates 1
month, present in heart, brain,
muscles, germinal centers at
least 3 weeks post injection
included in exosomes
circulates 4 months
LNPs
Feces, urine, saliva,
sweat, maternal milk,
unexplored in semen
mRNA naked
and in EVs in
human maternal
milk
Spike in EVs in
keratinocytes 3
months post
injection
Penetration of
vaccine products
mRNA and spike
circulate in LNPs or in natural EVs
that have been shown to penetrate
transdermally and by inhalation,
orally (breast milk) or by trans
placental route
Excretion
HYPOTHESIS
Infectious Diseases Research 2022;3(4):22. https://doi.org/10.53388/IDR20221125022
Submit a manuscript: https://www.tmrjournals.com/idr 6
transport material to target cells, an event that plays an important role
in responses to viral infections. SARS-CoV-2-S protein or derived
fragments were clearly present in the exosomes of COVID-19 patients.
SARS-CoV-2-S-derived fragments are present in exosomes from all
COVID-19 patients [64].
Spike also circulates in exosomes after mRNA vaccination in
humans. Authors have proposed that after LNP internalization and
mRNA release, antigen sorting and trafficking can induce the release
of S protein-containing exosomes. The events presented would occur
in the apical and/or basolateral surfaces of polarized (e.g. epithelial)
cells [65]. Indeed, the vaccine spike is spontaneously enveloped in
exosomes or extra-cellular vesicles: Vaccination with mRNA and
translation of the mRNA induces the production of exosomes carrying
the spike protein and circulating in the blood 14 days after injection
and up to 4 months after vaccination. Injection of these exosmoes into
mice induces the synthesis of anti-spike antibodies [66].
Vaccine spike was found in keratinocyte vesicles of dermal
endothelial cells from a patient with skin lesions 3 months after
vaccination with Pfizer-BioNTech vaccine. This patient had
varicella-zoster virus infection. A plausible hypothesis was that RNA
stabilization by methyl-pseudouridine substitution at all uridine
nucleotides for BNT162b2 could result in long-term production of the
encoded spike from any cell, persistently affecting the
microenvironment of the protective immune system, including the
skin [67].
All these data indicate that vaccine LNPs or exosomes naturally
formed after vaccination could contain mRNA or spike and could be
present in body fluids. Are these nanoparticles capable of entering
from these fluids into the bodies of unvaccinated individuals in
contact with freshly vaccinated individuals?
Ability of LNPs or natural extracellular vesicles (EVs or exosomes)
and mRNA to enter through different pathways
Use of nanoparticles for therapeutic purposes by inhalation,
transdermal, in utero, conjunctival route
In a review dedicated to the safety of nanoparticles in biomedical
applications, we learned that exposure to LNPs can occur through
ingestion, injection, inhalation and skin contact. Some exposures are
unintentional, such as pulmonary inhalation of NPs in the
environment or at manufacturing sites [68].
Nanosystems are increasingly being exploited for topical and dermal
delivery, including therapeutic peptides, proteins, vaccines, gene
fragments, or drug carrier particles [69]. Intradermal administration
of mRNA encoding vascular endothelial growth factor (VEGF) has
been shown to result in functional protein expression in the skin even
in the absence of lipid nanoparticles [70]. According to Palmer et al
[71], in a lipid nanoparticle formulation, liposomes increase the
transdermal passage of molecules used to treat skin diseases. Skin
penetration of siRNAs has been demonstrated in the form of
nano-carriers, these siRANs transfect cells and express the targeted
gene of interest. Nanocarriers have been tested for use in transdermal
vaccination [72].
Extracellular vesicles are used to deliver therapies other than
vaccines: clinical studies are underway by local route (periondontitis,
ulcers, epidermolysis bullosa) and by inhalation (ongoing trial against
Alzheimer's disease) [73]. Lipid nanoparticles with a lipid bilayer are
able to pass the skin barrier and carry genetic material. These particles
can penetrate the skin through hair follicles or directly into
keratinocytes due to their similarity to cell membranes [74].
Intranasal, oral, and intraocular and subconjunctival administration
of extracellular vesicles capable of carrying drugs has been
successfully tested.
Intranasal administration represents the second most frequently
reported route. It is effective in transporting drugs into the CNS, into
the lungs. Most of the protective effects were obtained in a similar way
for intravenous and intranasal administration. Oral administration has
been described for EVs from bovine milk in a mouse model. Six hours
after administration, vesicles were localized in the liver, heart, spleen,
lungs, and kidneys. Intraocular and subconjunctival injection of
MSC-derived EVs (stem cells) delivered vesicles to the retina in a
rabbit model of diabetes-induced retinopathy [75].
Nanovesicles naturally produced by plants are morphologically and
functionally identical to their mammalian analogues. A review on
plant nanovesicles brings together knowledge on the transdermal,
transmembrane, and targeting mechanisms of these vesicles.
Experiments on mice have shown that it is possible to deliver RNA
into a brain tumor via these intranasally introduced nanovesicles.
These nanovesicles would also be able to efficiently transport their
cargo through the skin and into the skin cells [76].
Lipid nanoparticles are a potential carrier for delivering molecules
to the posterior chamber of the eye: they have demonstrated excellent
ocular permeation characteristics and penetration-enhancing
capabilities, while exhibiting high drug loading and entrapment
efficiencies [77].
Nanoparticles in vaccination and gene therapy trials (LNPs
containing nucleic acids) via the respiratory route
Nucleic acid cargo nanoparticles are capable of transfecting airway
cells in animals and humans by local administration (instillation or
nebulization). The DEFUSE project [78], submitted by Eco Health
Alliance in response to a DARPA call for proposals, deals with the
transcutaneous administration of vaccines in animals using
nanoparticles. For therapeutic purposes, the LNPs formulation was
optimized for lung penetration by inhalation and it was verified that
mRNA is efficiently translated in the lung after nebulization (mouse
assay) [79].
The intranasal route has also been studied for vaccination with
mRNA cargo LNPs as well as for gene therapy in cystosis fibrosis with
mRNAs encapsulated in LNPs by the intranasal route by instillation in
the nostrils of mice: the mRNA transfects the nasal cells and expresses
the protein of interest in cells that did not express it because of a
genetic defect [80].
In humans, liposomal DNA-containing nanoparticles administered
locally by nebulization have transfected airway cells. A recent phase
2b trial of cystic fibrosis transmembrane conductance regulator
(CFTR) DNA delivery using a liposomal delivery system showed that
after repeated monthly nebulizations for one year, the cystic fibrosis
patient groups experienced a stabilization of lung function, while the
placebo group experienced a decline [81].
Clinical trials for influenza prevention have shown the efficacy and
safety of inhaled mRNA vaccines: Bare mRNA or mRNA enveloped in
lipid particles (especially PEG-based as in the anti-COVID mRNA
vaccines), is able to be inhaled in an aerosol and transfect lung
epithelial cells [82]. In utero administration of lipid nanoparticle
formulations containing mRNA can be applied to deliver mRNA to
mouse fetuses, resulting in protein expression in the fetal liver, lungs,
and intestines [70].
Testing LNPs for transcutaneous vaccination
In a review [72] on the possibility of transcutaneous vaccination with
LNPs, we learn that undamaged human skin is impermeable to microand
nanoparticles but there is evidence of some dermal penetration
into viable tissues (mainly in the stratum spinosum of the epidermal
layer, but also possibly in the dermis) for very small particles (less
than 10 nm). When using intact skin penetration protocols, there is no
conclusive evidence of skin penetration into viable tissues for particles
with a primary size of about 20 nm and larger. But there is no
information appropriate for skin with impaired barrier function, e.g.,
atopic skin or sunburned skin. Some data are available on psoriatic
skin. There is evidence that some mechanical effects (e.g., bending) on
the skin may affect nanoparticle penetration. But it has been shown
that nanoparticles accumulate in follicular openings, sebaceous glands
or skin folds. An aqueous suspension of nanoparticles as well as a
hydrogel formulation of these particles, applied to pig ear skin in
vitro, penetrated deep into the hair follicles. These particles can
release various encapsulated compounds that then penetrate the skin.
There is evidence in the literature that the trans-follicular route can be
HYPOTHESIS
Infectious Diseases Research 2022;3(4):22. https://doi.org/10.53388/IDR20221125022
Submit a manuscript: https://www.tmrjournals.com/idr 7
used: topical application of naked plasmid expression vectors to intact
mouse skin induced antigen-specific immune responses.
HBsAg-specific cellular and antibody responses were induced in the
same order of magnitude as those produced by intramuscular (IM)
injection of recombinant HBsAg polypeptide vaccine. In contrast, no
immune response could be induced in nude mice: the presence of
normal hair follicles was a prerequisite for the induction of a response.
The particles were approximately 150 nm in size. LNPs in mRNA
vaccines are between 100 and 400 nm [22].
One system that has been clinically tested transdermally is the
DermaVir HIV-1/AIDS patch. It contains a plasmid DNA (pDNA)
vaccine encoding all major HIV-1 antigens and viral-like particle
formation.) The pDNA is formulated as mannosylated
polyethyleneimine nanoparticles (80-400 nm) similar to those of
pathogens. This study involved 12 people immunized with the
vaccine: they developed higher and broader levels of CD8+ T cells
compared to placebo, although it had no effect on CD4+ T cell
numbers [72].
Naked RNA could also be used via skin passage and inhalation
RNA oligonucleotides can penetrate intact skin and retain their
biological activity, penetration through the skin does not depend on
the size of the molecule under study (12.5 to 29.3 kDA) [83].
The feasibility of inhaled RNA for passive transfection has also been
demonstrated in a number of studies. Mechanistically, inhaled RNA
can lead to passive synthesis of non-infectious spike proteins using cell
transfection machines, thus leading to immunization of the individual
[84].
Therapeutic and vaccine LNPs in COVID-19
Given that vaccine LNPs are synthetic exosomes it is not surprising
that COVID therapeutics and vaccines with natural exosomes as
vectors are being tested. Nebulization of exosomes for inhalation
therapy has been tested against COVID-19. Clinical trials are
underway to deliver aerosolized anti-viral therapies in EVs in
COVID-19. Currently, over sixty clinical trials are underway to study
the effects of MSCs (mesenchymal stem cells) and EVs (containing
these MSCs) in COVID-19 patients. A phase 1 clinical trial to evaluate
the safety and efficacy of inhaled exosomes derived from allogeneic
adipose MSCs for the treatment of COVID-19 pneumonia has been
completed. 3 clinical trials used aerosol as the route of administration
[16]. In 2022, MSCs exosomes showed efficacy for nebulization
therapy in COVID-19 patients [85].
Natural exosome vaccines against SARS-CoV-2: plantar or
inhalation route
Exosome vaccines carrying mRNA have been considered against
SARS-CoV-2 [86]. Vaccine trials injected as exosomes into the footpad
of mice showed induction of antibodies against spike [87]. Spike RBD
exosomes (nanoparticles) are capable of nebulizing and inhaling
antigen into mouse lung cells and inducing an immune response. They
are virus-like particles (virus like particles (VLP)) naturally obtained
from lung cells and carry RNA from their parent cell as well as various
proteins expressed on their surface [88]. Also by inhalation, exosomes
containing mRNA or spike protein are able to immunize mice or
non-human primates against SARS-CoV-2 and natural EVs are more
effective than synthetic EVs [89].
The possible reinterpretation of a study may support vaccine
shedding
Scientists compared unvaccinated children living with unvaccinated
parents with children who were also unvaccinated but living with
vaccinated parents [90]. The children of vaccinated parents have
anti-COVID IgG in their nose and the difference with the children of
unvaccinated parents is significant. The authors think that this is due
to antibody shedding by droplets: what is transferred would be the IgG
antibody itself in the saliva droplets. But it is possible that children
develop intranasal IgG because other vaccine byproducts or exosomes
are excreted by their vaccinated parents. This could be due to lipid
nanoparticles of mRNA that could be excreted and transferred through
saliva, sputum or skin. Children would develop an immune response
to the nanoparticles (or vaccine by-products) instead of IgG being
transferred directly from parents to children. The antibodies sought
are IgG and IgA against the RBD of the spike and not against the
nucleocapsid of the virus, which is a pity because the authors have
developed both types of tests [91]: this does not allow to distinguish
children who would have been naturally infected by the virus (and
would have anti-RBD and anti-N antibodies) from children who would
have developed antibodies following their parents' vaccination (and
would only have anti-RBD and no anti-N because not induced by the
vaccine).
Conclusion
There are many testimonies of non-vaccinated persons who
experienced symptoms identical to the adverse effects of the vaccine
after having been in contact with freshly vaccinated persons. A study
shows an excess of mortality in the non-vaccinated age groups when
vaccination campaigns begin, which could be explained by a
phenomenon of transmission of the vaccine or its products.
It is important not to neglect these testimonies because the required
studies of pharmacokinetics and in particular of excretion of the
vaccine and its products have not been carried out in spite of the
regulations in force for gene therapies, which include mRNA vaccines
according to the definition of these gene products. Moreover, the
doubt about the possible transmission of the vaccine creates an
unhealthy climate of suspicion of the non-vaccinated towards the
vaccinated: a clarification would therefore be welcome.
The vaccines are all based on the spike protein, which has since
been recognized as the main responsible for the pathogenicity of the
SARS-CoV-2 virus: if transmission of the vaccine or of the spike is
possible, it is logical to find the adverse effects of the vaccine in
non-vaccinated people in contact with vaccinated people.
Little is known about the pharmacokinetics of the vaccine. Vaccine
LNPs are very similar to natural EVs or exosomes, whose structure and
function scientists have tried to mimic as closely as possible.
According to the few studies conducted by manufacturers and
independent researchers, mRNA vaccine LNPs circulate in the blood
and accumulate in the spleen and liver of mice (and to a lesser extent
in many organs including ovaries and testes, bone marrow,..).
Translation into spike protein persists 6 to 10 days in mice at the
injection site and 8 days in the muscles.
The route of excretion of LNPs varies according to their size, in the
case of LNPs of mRNA vaccines excretion should be mainly by the
feces but also by the urine. The quantitative results of these studies
suggest that other routes of excretion than feces and urine should be
explored. Studies prior to mRNA vaccines suggest that EV excretion is
possible through saliva, sweat, and breast milk.
Studies have shown that it is very possible that nanoparticles of
comparable size to those used for mRNA vaccines are capable of
transplacental passage in humans. Natural nanoparticles (EVs) are
naturally present in all body fluids (including sputum, saliva, and
sweat) and in keratinocytes and can carry nucleic acids that are thus
protected from nucleases. Certain types of RNA (miRNAs) are
selectively selected and enriched in sweat EVs from blood. No studies
have been found regarding the possibility of passage of LNPs into
semen; given the biodistribution in all organs and fluids, such passage
is a priori possible and should be explored.
Viral RNA of many viruses is found in blood, secretions and tissues.
Vaccine mRNA is injected in quantities orders of magnitude greater
than the viral RNA produced during natural infection. This mRNA is
found in the blood as early as the first day after injection and persists
for up to 15 days. It is able to escape from LNPs and to be
encapsulated in EVs, it is functional and can be translated into protein.
Vaccine mRNA naked or encapsulated in EVs is found in breast milk
within the first week after injection; it is protected from gastric juices
and can transfect neonatal cells.
HYPOTHESIS
Infectious Diseases Research 2022;3(4):22. https://doi.org/10.53388/IDR20221125022
Submit a manuscript: https://www.tmrjournals.com/idr 8
RNA embedded in EVs or even naked is capable of transfecting cells
by inhalation or transdermal passage. Intranasal, oral, transdermal
intraocular and subconjunctival administration of extracellular
drug-carrying vesicles has been tested: LNPs can be administered
through the skin, intranasally, intraconjunctivally and by inhalation;
experiments have shown that mRNA included in these LNPs is capable
of transfecting cells Vaccination trials against COVID by inhalation of
EVs containing mRNA or spike protein have shown positive results in
mice and nonhuman primates. Natural EVs are more effective than
synthetic EVs.
Spike protein translated from vaccine mRNA persists for months in
large quantities in vaccinees; it is found in free form in plasma and
encapsulated in EVs that form spontaneously from the cells where
spike was produced. These EVs can deliver their cargo to different cell
types, in particular to fetal cells of vaccinated mothers. Spike can be
found in keratinocytes of the skin.
Specifically against coronaviruses, gene therapy and vaccination
trials (especially with mRNA) have shown the possibility of
transfecting cells transcutaneously, nasally and by nebulization from
LNPs and even from naked mRNA. Spike or mRNA RBD vector
exosomes have been tested by inhalation in animals for anti-COVID-19
immunization.
All these studies show that EVs carrying mRNA and spike could
therefore be excreted by different body fluids and could enter by
transcutaneous or inhalation route in unvaccinated individuals (as
well as by breast milk in infants and by transplacental passage in
fetuses and why not by semen). Naked mRNA could also be excreted
and entered.
The mRNA (and adenovirus) vaccines correspond exactly to the
definition of gene therapy given by the health agencies (FDA, NIH and
EMA). According to the regulations of these agencies, these products
should be subject to additional pharmacokinetic studies (in particular
excretion studies) as a matter of urgency as the widespread use of
mRNA technology becomes apparent. Indeed, Sanofi launched clinical
trial of the first mRNA-based seasonal flu vaccine candidate [92],
Moderna launched phase 3 trial of mRNA influenza vaccine [93]. For
these flu vaccines, emergency approval should not be applied and the
requirement for these additional studies should not be exceeded.
References
1. Covid Vaccine Side Effects Ray Sahelian.
https://raysahelian.com/covidvaccinesideeffects.html Accessed
November 3, 2022
2. Moghaddar M, Radman R, Macreadie I. Severity, pathogenicity
and transmissibility of delta and lambda variants of
SARS-CoV-2, toxicity of spike protein and possibilities for future
prevention of COVID-19. Microorganisms 2021;9(10):2167.
http://doi.org/10.3390/microorganisms9102167
3. Almehdi AM, Khoder G, Alchakee AS, Alsayyid AT, Sarg NH,
Soliman SSM. SARS-CoV-2 spike protein: pathogenesis, vaccines,
and potential therapies. Infection 2021;49(5):855-876.
http://doi.org/10.1007/s15010-021-01677-8
4. Lei Y, Zhang J, Schiavon CR, et al. SARS-CoV-2 spike protein
impairs endothelial function via downregulation of ACE 2. Circ
Res 2021;128(9):1323-1326.
http://doi.org/10.1161/CIRCRESAHA.121.318902
5. Letarov AV, Babenko VV, Kulikov EE. Free SARS-CoV-2 spike
protein S1 particles may play a role in the pathogenesis of
COVID-19 infection. Biochemistry Moscow 2020;86(3):257-261.
http://doi.org/10.1134/S0006297921030032
6. Gao X, Zhang S, Gou J, et al. Spike-mediated ACE2
down-regulation was involved in the pathogenesis of
SARS-CoV-2 infection. J Infection 2022;85(4):418-427.
http://doi.org/10.1016/j.jinf.2022.06.030
7. Coronavirus vaccine - summary of Yellow Card reporting.
https://www.gov.uk/government/publications/coronavirus-co
vid-19-vaccine-adverse-reactions/coronavirus-vaccine-summar
y-of-yellow-card-reporting#annex-1-vaccine-analysis-print
Accessed November 3, 2022
8. Pantazakos S, Seligmann H. COVID vaccination and
age-stratified all-cause mortality risk.
https://www.researchgate.net/publication/355581860_COVID_
vaccination_and_age-stratified_all-cause_mortality_risk Accessed
2021
9. Internet Archives.
https://archive.org/details/pfizer-confidential-translated
SARS-CoV-2 mRNA Vaccine (BNT162, PF-07302048) 2.6.4
Summary statement of the pharmacokinetic
studyhttps://ia902305.us.archive.org/28/items/pfizer-confide
ntial-translated/pfizer-confidential-translated.pdf Accessed
November 3, 2022
10. Design and analysis of shedding studies for virus or
bacteria-based gene therapy and oncolytic products.
https://www.fda.gov/regulatory-information/search-fda-guida
nce-documents/design-and-analysis-shedding-studies-virus-or-b
acteria-based-gene-therapy-and-oncolytic-products Accessed
November 3, 2022
11. NIH GUIDELINES FOR RESEARCH INVOLVING RECOMBINANT
OR SYNTHETIC NUCLEIC ACID MOLECULES (NIH
GUIDELINES) APRIL 2019
https://osp.od.nih.gov/wp-content/uploads/NIH_Guidelines.pd
f Accessed November 3, 2022
12. mRNA Clinical trials: key regulatory considerations.
https://www.advarra.com/blog/mrna-clinical-trials-key-regula
tory-considerations/ Accessed November 3, 2022
13. European Medicines Agency. Guideline on the quality,
non-clinical and clinical aspects of gene therapy medicinal
products.
https://www.ema.europa.eu/en/documents/scientific-guidelin
e/guideline-quality-non-clinical-clinical-aspects-gene-therapymedicinal-
products_en.pdf Accessed November 3, 2022
14. Committee for Medicinal Products for Human Use (CHMP)
Assessment report , Onpattro , International non-proprietary
name: patisiran Procedure No. EMEA/H/C/004699/0000.
https://www.ema.europa.eu/en/documents/assessment-report
/onpattro-epar-public-assessment-report_.pdf Published July 26,
2018 Accessed November 3, 2022
15. Pfizer. A phase 1/2/3, placebo controlled, randomized,
observer-blind, dose-finding study to evaluate the safety,
tolerability, immunogenicity, and efficacy of SARS-CoV-2 RNA
vaccine candidates against Covid-19 in healthy individuals.
https://cdn.pfizer.com/pfizercom/2020-11/C4591001_Clinical_
Protocol_Nov2020.pdf Accessed November 3, 2022
16. Machhi J, Shahjin F, Das S, et al. A role for extracellular vesicles
in SARS-CoV-2 therapeutics and prevention. J Neuroimmune
Pharmacol 2021;16(2):270-288.
http://doi.org/10.1007/s11481-020-09981-0
17. Aday S, Hazan-Halevy I, Chamorro-Jorganes A, et al.
Bioinspired artificial exosomes based on lipid nanoparticles
carrying let-7b-5p promote angiogenesis in vitro and in vivo.
Mol Ther 2021;29(7):2239-2252.
http://doi.org/10.1016/j.ymthe.2021.03.015
18. Exosomes - The Good, Bad, Ugly and Current State.
https://www.americanpharmaceuticalreview.com/Featured-Ar
ticles/575432-Exosomes-The-Good-Bad-Ugly-and-Current-State
/ Published Apri 26, 2021 Accessed November 3, 2022
19. Pilkington EH, Suys EJA, Trevaskis NL, et al. From influenza to
COVID-19: lipid nanoparticle mRNA vaccines at the frontiers of
infectious diseases. Acta Biomater 2021;131:16-40.
http://doi.org/10.1016/j.actbio.2021.06.023
20. Schoenmaker L, Witzigmann D, Kulkarni JA, et al. mRNA-lipid
nanoparticle COVID-19 vaccines: structure and stability. Int J
Pharm 2021;601:120586.
http://doi.org/10.1016/j.ijpharm.2021.120586
21. Shepherd MC, Radnaa E, Tantengco OA, et al. Extracellular
vesicles from maternal uterine cells exposed to risk factors
cause fetal inflammatory response. Cell Commun Signal
HYPOTHESIS
Infectious Diseases Research 2022;3(4):22. https://doi.org/10.53388/IDR20221125022
Submit a manuscript: https://www.tmrjournals.com/idr 9
2021;19(1):100.
http://doi.org/10.1186/s12964-021-00782-3
22. Di J, Du Z, Wu K, et al. Biodistribution and non-linear gene
expression of mRNA LNPs affected by delivery route and
particle size. Pharm Res 2022;39(1):105-114.
http://doi.org/10.1007/s11095-022-03166-5
23. Brohi RD, Wang L, Talpur HS, et al. Toxicity of nanoparticles on
the reproductive system in animal models: a review. Front
Pharmacol 2017;8:606.
http://doi.org/10.3389/fphar.2017.00606
24. Li M, Al-Jamal KT, Kostarelos K, Reineke J. Physiologically
based pharmacokinetic modeling of nanoparticles. ACS Nano
2010;4(11):6303-6317.
http://doi.org/10.1021/nn1018818
25. Zhao Y, Sultan D, Liu Y. 2-Biodistribution, excretion, and
toxicity of nanoparticles. Theranostic Bionanomaterials
2019:27-53.
http://doi.org/10.1016/B978-0-12-815341-3.00002-X
26. Griffin DE. Why does viral RNA sometimes persist after
recovery from acute infections? PLoS Biol
2022;20(6):e3001687.
http://doi.org/10.1371/journal.pbio.3001687
27. Les vaccins ARN messagers (ARNm) sont-ils surdoss ?
https://tkp.at/wp-content/uploads/2022/05/Jean-Michel-Clav
erie.pdf Accessed November 3, 2022
28. Fertig TE, Chitoiu L, Marta DS, et al. Vaccine mRNA can be
detected in blood at 15 days post-vaccination. Biomedicines
2022;10(7):1538.
http://doi.org/10.3390/biomedicines10071538
29. Maugeri M, Nawaz M, Papadimitriou A, et al. Linkage between
endosomal escape of LNP-mRNA and loading into EVs for
transport to other cells. Nat Commun 2019;10(1):4333.
http://doi.org/10.1038/s41467-019-12275-6
30. Bart G, Fischer D, Samoylenko A, et al. Characterization of
nucleic acids from extracellular vesicle-enriched human sweat.
BMC Genomics 2021;22(1)425.
http://doi.org/10.1186/s12864-021-07733-9
31. Reverse engineering the source code of the BioNTech/Pfizer
SARS-CoV-2 vaccine.
https://berthub.eu/articles/posts/reverse-engineering-source-c
ode-of-the-biontech-pfizer-vaccine/ Published December 25,
2020 Accessed November 3, 2022
32. Karvinen S, Sievänen T, Karppinen JE, et al. MicroRNAs in
extracellular vesicles in sweat change in response to endurance
exercise. Front Physiol 2020;11:676.
http://doi.org/10.3389/fphys.2020.00676
33. Package leaflet: information for the recipient , COVID-19 mRNA
vaccine BNT162b2 concentrate for solution for injection
tozinameran.
https://assets.publishing.service.gov.uk/government/uploads/s
ystem/uploads/attachment_data/file/1043779/Temporary_Aut
horisation_Patient_Information_BNT162_18_0_UK_Clean.pdf
Accessed November 3, 2022
34. Should I sweat after my COVID vaccine ?
https://wickedsheets.com/night-sweats/should-i-sweat-after-m
y-covid-vaccine/ Published June 1, 2021 Accessed November 3,
2022
35. Shao S, Fang H, Li Q, Wang G. Extracellular vesicles in
inflammatory skin disorders: from pathophysiology to
treatment. Theranostics 2020;10(22):9937-9955.
http://doi.org/10.7150/thno.45488
36. Bar-Shai A, Rotem M, Shenhar-Tsarfaty S, Ophir N, Fireman E.
Nanoparticles in sputum - a new window to airway
inflammation. Eur Respir J 2017;50:PA1008.
http://doi.org/10.1183/1393003.congress-2017.PA1008
37. Sánchez-Vidaurre S, Eldh M, Larssen P, et al. RNA-containing
exosomes in induced sputum of asthmatic patients. J Allergy Clin
Immunol 2017;140(5):1459-1461.
http://doi.org/10.1016/j.jaci.2017.05.035
38. Low JM, Gu Y, Ng MSF, et al. Codominant IgG and IgA
expression with minimal vaccine mRNA in milk of BNT162b2
vaccinees. NPJ Vaccines 2021;6(1):105.
http://doi.org/10.1038/s41541-021-00370-z
39. New growth curves for French boys, French Association of
Ambulatory Pediatrics, Nouvelles courbes de croissance des
garçons français, Association Française de Pdiatrie
Ambulatoire.
https://afpa.org/outil/courbes-de-croissance-garcons-francais/
Accessed November 3, 2022
40. Golan Y, Prahl M, Cassidy A, et al. Evaluation of messenger RNA
from COVID-19 BTN162b2 and mRNA-1273 vaccines in human
milk. JAMA Pediatr 2021;175(10):1069.
http://doi.org/10.1001/jamapediatrics.2021.1929
41. Golan Y, Prahl M, Cassidy AG, et al. COVID-19 mRNA
vaccination in lactation: assessment of adverse events and
vaccine related antibodies in mother-infant dyads. Front
Immunol 2021;12:777103.
http://doi.org/10.3389/fimmu.2021.777103
42. Hanna N, Heffes-Doon A, Lin X, et al. Detection of messenger
RNA COVID-19 vaccines in human breast milk. JAMA Pediatr
Published online September 26, 2022.
http://doi.org/10.1001/jamapediatrics.2022.3581
43. Kahn S, Liao Y, Du X, Xu W, Li J, Lönnerdal B. Exosomal
microRNAs in milk from mothers delivering preterm infants
survive in vitro digestion and are taken up by human intestinal
cells. Mol Nutr Food Res 2018;62(11):1701050.
http://doi.org/10.1002/mnfr.201701050
44. Lonnerdal B, Du X, Liao Y, Li J. Human milk exosomes resist
digestion in vitro and are internalized by human intestinal cells.
FASEB J 2015;29(S1).
http://doi.org/10.1096/fasebj.29.1_supplement.121.3
45. Melnik BC, Schmitz G. Milk exosomal microRNAs: postnatal
promoters of β cell proliferation but potential inducers of β cell
de-differentiation in adult life. Int J Mol Sci 2022;23(19):11503.
http://doi.org/10.3390/ijms231911503
46. Riley RS, Kashyap MV, Billingsley MM, et al. Ionizable lipid
nanoparticles for in utero mRNA delivery. Sci Adv
2021;7(3):eaba1028.
http://doi.org/10.1126/sciadv.aba1028
47. LaTourette PC II, Awasthi S, Desmond A, et al. Protection
against herpes simplex virus type 2 infection in a neonatal
murine model using a trivalent nucleoside-modified mRNA in
lipid nanoparticle vaccine. Vaccine 2020;38(47):7409-7413.
http://doi.org/10.1016/j.vaccine.2020.09.079
48. Kulvietis V, Zalgeviciene V, Didziapetriene J, Rotomskis R.
Transport of nanoparticles through the placental barrier.
Tohoku J Exp Med 2011;225(4):225-234.
http://doi.org/10.1620/tjem.225.225
49. Saunders M. Transplacental transport of nanomaterials. WIREs:
Nanmed Nanobiotech 2009;1(6):671-684.
http://doi.org/10.1002/wnan.53
50. Muoth C, Aengenheister L, Kucki M, Wick P, Buerki-Thurnherr
T. Nanoparticle transport across the placental barrier: pushing
the field forward! Nanomedicine 2016;11(8):941-957.
http://doi.org/10.2217/nnm-2015-0012
51. Keelan JA, Leong JW, Ho D, Iyer KS. Therapeutic and safety
considerations of nanoparticle-mediated drug delivery in
pregnancy. Nanomedicine 2015;10(14):2229-2247.
http://doi.org/10.2217/nnm.15.48
52. Wick P, Malek A, Manser P, et al. Barrier capacity of human
placenta for nanosized materials. Environ Health Perspect
2010;118(3):432-436.
http://doi.org/10.1289/ehp.0901200
53. Bertozzi S, Corradetti B, Seriau L, et al. Nanotechnologies in
obstetrics and cancer during pregnancy: a narrative review. J
Pers Med 2022;12(8):1324.
http://doi.org/10.3390/jpm12081324
54. Soininen SK, Repo JK, Karttunen V, Auriola S, Vähäkangas KH,
HYPOTHESIS
Infectious Diseases Research 2022;3(4):22. https://doi.org/10.53388/IDR20221125022
Submit a manuscript: https://www.tmrjournals.com/idr 10
Ruponen M. Human placental cell and tissue uptake of
doxorubicin and its liposomal formulations. Toxicol Lett
2015;239(2):108-114.
http://doi.org/10.1016/j.toxlet.2015.09.011
55. Falchi L, Khalil WA, Hassan M, Marei WFA. Perspectives of
nanotechnology in male fertility and sperm function. Int J Vet
Sci Med 2018;6(2):265-269.
http://doi.org/10.1016/j.ijvsm.2018.09.001
56. SARS-CoV-2 mRNA Vaccine (BNT162, PF-07302048) 2.6.4
Summary statement of the pharmacokinetic study.
https://ia902305.us.archive.org/28/items/pfizer-confidential-t
ranslated/pfizer-confidential-translated.pdf Accessed November
4, 2022
57. Vaccine FAQ, CDC and IDSA.
https://www.idsociety.org/covid-19-real-time-learning-networ
k/vaccines/vaccines-information--faq/ Accessed November 4,
2022
58. Pardi N, Tuyishime S, Muramatsu H, et al. Expression kinetics of
nucleoside-modified mRNA delivered in lipid nanoparticles to
mice by various routes. J Control Release 2015;217:345-351.
http://doi.org/10.1016/j.jconrel.2015.08.007
59. Cosentino M, Marino F. The spike hypothesis in
vaccine-induced adverse effects: questions and answers. Trends
Mol Med 2022;28(10):797-799.
http://doi.org/10.1016/j.molmed.2022.07.009
60. Ogata AF, Maley AM, Wu C, et al. Ultra-sensitive serial profiling
of SARS-CoV-2 antigens and antibodies in plasma to understand
disease progression in COVID-19 patients with severe disease.
Clin Chem 2020;66(12):1562-1572.
http://doi.org/10.1093/clinchem/hvaa213
61. Ogata AF, Cheng CA, Desjardins M, et al. Circulating severe
acute respiratory syndrome coronavirus 2 (SARS-CoV-2)
vaccine antigen detected in the plasma of mRNA-1273 vaccine
recipients. Clin Infect Dis 2021;74(4):715-718.
http://doi.org/10.1093/cid/ciab465
62. Cognetti JS, Miller BL. Monitoring serum spike protein with
disposable photonic biosensors following SARS-CoV-2
vaccination. Sensors 2021;21(17):5857.
http://doi.org/10.3390/s21175857
63. Mörz M. A case report: multifocal necrotizing encephalitis and
myocarditis after BNT162b2 mRNA vaccination against
COVID-19. Vaccines 2022;10(10):1651.
http://doi.org/10.3390/vaccines10101651
64. Pesce E, Manfrini N, Cordiglieri C, et al. Exosomes recovered
from the plasma of COVID-19 patients expose SARS-CoV-2
spike-derived fragments and contribute to the adaptive immune
response. Front Immunol 2022;12:785941.
http://doi.org/10.3389/fimmu.2021.785941
65. Trougakos IP, Terpos E, Alexopoulos H, et al. Adverse effects of
COVID-19 mRNA vaccines: the spike hypothesis. Trends Mol
Med 2022;28(7):542-554.
http://doi.org/10.1016/j.molmed.2022.04.007
66. Bansal S, Perincheri S, Fleming T, et al. Cutting edge:
circulating exosomes with COVID spike protein are induced by
BNT162b2 (Pfizer–BioNTech) vaccination prior to development
of antibodies: a novel mechanism for immune activation by
mRNA vaccines. J Immunol 2021;207(10):2405-2410.
http://doi.org/10.4049/jimmunol.2100637
67. Yamamoto M, Kase M, Sano H, Kamijima R, Sano S. Persistent
varicella zoster virus infection following mRNA COVID-19
vaccination was associated with the presence of encoded spike
protein in the lesion. J Cutan Immunol Allergy 2022;00:1-6.
https://doi.org/10.1002/cia2.12278
68. Najahi-Missaoui W, Arnold RD, Cummings BS. Safe
nanoparticles: are we there yet? Int J Mol Sci 2021;22(1):385.
http://doi.org/10.3390/ijms22010385
69. Roberts M, Mohammed Y, Pastore M, et al. Topical and
cutaneous delivery using nanosystems. J Control Release
2017;247:86-105.
http://doi.org/10.1016/j.jconrel.2016.12.022
70. Hou X, Zaks T, Langer R, Dong Y. Lipid nanoparticles for mRNA
delivery. Nat Rev Mater 2021;6(12):1078-1094.
http://doi.org/10.1038/s41578-021-00358-0
71. Palmer B, DeLouise L. Nanoparticle-enabled transdermal drug
delivery systems for enhanced dose control and tissue targeting.
Molecules 2016;21(12):1719.
http://doi.org/10.3390/molecules21121719
72. Hansen S, Lehr CM. Nanoparticles for transcutaneous
vaccination. Microb Biotechnol 2011;5(2):156-167.
http://doi.org/10.1111/j.1751-7915.2011.00284.x
73. Klyachko NL, Arzt CJ, Li SM, Gololobova OA, Batrakova EV.
Extracellular vesicle-based therapeutics: preclinical and clinical
investigations. Pharmaceutics 2020;12(12):1171.
http://doi.org/10.3390/pharmaceutics12121171
74. Gu TW, Wang MZ, Niu J, Chu Y, Guo KR, Peng LH. Outer
membrane vesicles derived from E. colias novel vehicles for
transdermal and tumor targeting delivery. Nanoscale
2020;12(36):18965-18977.
http://doi.org/10.1039/D0NR03698F
75. De Jong B, Barros ER, Hoenderop JGJ, Rigalli JP. Recent
advances in extracellular vesicles as drug delivery systems and
their potential in precision medicine. Pharmaceutics
2020;12(11):1006.
http://doi.org/10.3390/pharmaceutics12111006
76. Dad HA, Gu TW, Zhu AQ, Huang LQ, Peng LH. Plant
exosome-like nanovesicles: emerging therapeutics and drug
delivery nanoplatforms. Mol Ther 2021;29(1):13-31.
http://doi.org/10.1016/j.ymthe.2020.11.030
77. Jacob S, Nair AB, Shah J, et al. Lipid nanoparticles as a
promising drug delivery carrier for topical ocular therapy—an
overview on recent advances. Pharmaceutics 2022;14(3):533.
http://doi.org/10.3390/pharmaceutics14030533
78. The DEFUSE PROJECT Documents.
https://drasticresearch.org/2021/09/21/the-defuse-project-doc
uments/ Accessed November 4, 2022
79. Zhang H, Leal J, Soto MR, Smyth HDC, Ghosh D. Aerosolizable
lipid nanoparticles for pulmonary delivery of mRNA through
design of experiments. Pharmaceutics 2020;12(11):1042.
http://doi.org/10.3390/pharmaceutics12111042
80. Robinson E, MacDonald KD, Slaughter K, et al. Lipid
nanoparticle-delivered chemically modified mRNA restores
chloride secretion in cystic fibrosis. Mol Ther
2018;26(8):2034-2046.
http://doi.org/10.1016/j.ymthe.2018.05.014
81. Alton EWFW, Armstrong DK, Ashby D, et al. Repeated
nebulisation of non-viral CFTR gene therapy in patients with
cystic fibrosis: a randomised, double-blind, placebo-controlled,
phase 2b trial. Lancet Respir 2015;3(9):684-691.
http://doi.org/10.1016/S2213-2600(15)00245-3
82. Chow MYT, Qiu Y, Lam JKW. Inhaled RNA therapy: from
promise to reality. Trends Pharmacol Sci 2020;41(10):715-729.
http://doi.org/10.1016/j.tips.2020.08.002
83. Lenn JD, Neil J, Donahue C, et al. RNA aptamer delivery
through intact human skin. J Invest Dermatol
2018;138(2):282-290.
http://doi.org/10.1016/j.jid.2017.07.851
84. Yeo WS, Ng QX. Passive inhaled mRNA vaccination for
SARS-Cov-2. Med Hypotheses 2021;146:110417.
http://doi.org/10.1016/j.mehy.2020.110417
85. Chu M, Wang H, Bian L, et al. Nebulization therapy with
umbilical cord mesenchymal stem cell-derived exosomes for
COVID-19 pneumonia. Stem Cell Rev Rep 2022;18(6):2152-2163.
http://doi.org/10.1007/s12015-022-10398-w
86. Sabanovic B, Piva F, Cecati M, Giulietti M. Promising
extracellular vesicle-based vaccines against viruses, including
SARS-CoV-2. Biology 2021;10(2):94.
http://doi.org/10.3390/biology10020094
87. Kuate S, Cinatl J, Doerr HW, Überla K. Exosomal vaccines
HYPOTHESIS
Infectious Diseases Research 2022;3(4):22. https://doi.org/10.53388/IDR20221125022
Submit a manuscript: https://www.tmrjournals.com/idr 11
containing the S protein of the SARS coronavirus induce high
levels of neutralizing antibodies. Virology 2007;362(1):26-37.
http://doi.org/10.1016/j.virol.2006.12.011
88. Wang Z, Popowski KD, Zhu D, et al. Exosomes decorated with a
recombinant SARS-CoV-2 receptor-binding domain as an
inhalable COVID-19 vaccine. Nat Biomed Eng
2022;6(7):791-805.
http://doi.org/10.1038/s41551-022-00902-5
89. Popowski KD, Moatti A, Scull G, et al. Inhalable dry powder
mRNA vaccines based on extracellular vesicles. Matter
2022;5(9):2960-2974.
http://doi.org/10.1016/j.matt.2022.06.012
90. Kedl RM, Hsieh E, Morrison TE, et al. Evidence for aerosol
transfer of SARS-CoV2-specific humoral immunity. medRxiv
https://doi.org/10.1101/2022.04.28.22274443
91. Schultz JS, McCarthy MK, Rester C, et al. Development and
validation of a multiplex microsphere immunoassay using dried
blood spots for SARS-CoV-2 seroprevalence: application in first
responders in Colorado, USA. J Clin Microbiol
2021;59(6):e00290-21.
http://doi.org/10.1128/JCM.00290-21
92. Sanofi launches clinical trial of first mRNA-based seasonal flu
vaccine candidate (Sanofi lance l'essai clinique du premier
candidat-vaccin base d'ARNm contre la grippe saisonnière)
https://www.capital.fr/entreprises-marches/sanofi-lance-lessaiclinique-
du-premier-candidat-vaccin-a-base-darnm-contre-la-gri
ppe-1407163 Accessed November 4, 2022
93. Moderna takes mRAN influenza candidate into Phase 3 trials.
https://www.biopharma-reporter.com/Article/2022/06/07/m
oderna-takes-mrna-influenza-candidate-into-phase-3-trial
Accessed November 4, 2022